Here’s a challenge: envision a trillionth of something. You might think of one penny compared to 10 billion dollars. Or how in travelling one meter, the Earth completes around a trillionth of its annual journey. Now you can add one more thing to the list, thanks to researchers in Zurich: a picometer, or a trillionth of a meter, is around the smallest distance that humans can resolve with a microscope.
Building on their impressive microscopy work over the last few years, a team from IBM has refined their method to precisely measure the structural details of a single molecule. With their technique, they managed to measure very subtle differences in the distribution of electrons within the molecule’s bonds. How subtle? We’re talking 3 picometers or 0.000000000003 meters.
That’s one-hundredth the diameter of an atom!
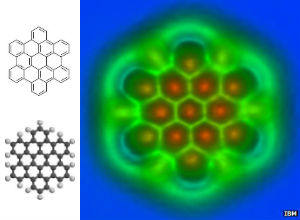
Having the ability to resolve the internal structure of a molecule at that level of precision is unprecedented. It validates decades of theoretical and indirect experimental work about the internal nature of molecules as well as promises a level of mastery in understanding the molecular world that could usher in nanoscale engineering. Their research was reported in Science magazine.
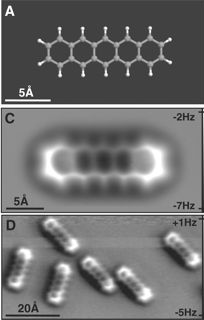
The IBM researchers add this latest accomplishment to a growing list of impressive microscopy feats. Three years ago, they reported the first high resolution image of a single molecule called pentacene, showing actual molecular bonds with clarity. That same year they precisely measured the electric charge of a single atom using a tuning fork-like device. Then earlier this year, the researchers announced that the distribution of electric charges on a single molecule, which had been theoretically described for years, was experimentally verified with their technique. In honor of this year’s Olympics, the team also imaged the molecule olympicene, which like pentacene consists of five fused rings but have the arrangement of the Olympic rings logo.
To truly appreciate the implications of this research, it’s worth digging a little deeper into this experiment.
The molecule pictured above, hexabenzocoronene (HBC), is a polyaromatic hydrocarbon that is planar, as where the other molecules the team has studied. That means HBC is a giant flat disc packed with electrons consisting of carbon atoms (except for the hydrogens around the edge).
Now a carbon atom bonds to another carbon atom by sharing electrons. The more electrons they share, the closer the nuclei of the atoms are to one another. So, if you know the precise length of a bond, you can better understand the electronic properties of those atoms. The new technique determined that the chemical bonds in the middle of the molecule were slightly shorter than ones near the edge. This indicated that these bonds have more electrons present.
HBC is of interest because it’s been used as a model to better understand the cool properties of graphene carbon sheets, carbon spheres called buckyballs, and carbon nanotubes. Furthermore, HBCs like to self assemble into large columnar stacks under the right conditions, making them a candidate for nanotechnology applications.
Because the team is able to measure bond lengths to within 3 picometers, they have a much more accurate picture of the electron properties throughout these molecule. They’ve even started testing other carbon grid molecules to see how the electronic properties of the whole molecule become distorted by other kinds of atoms or irregularities.
Why is that important? Because the electronic properties of this lattice is what makes gives carbon nanotubes their superconductive properties and sensor abilities. Understanding how even the littlest changes can cause electrons to change the way they are distributed in the molecule is of utmost importance if carbon nanotubes are going to useful and reliable in future technologies.
The technique developed by IBM uses atomic force microscopy or AFM with a single carbon monoxide (CO) at the tip that interacts with the molecule being probed. Sensitive interactions can be measured so, as the authors state, “The CO termination of the tip acts as a powerful magnifying glass to reveal the atomic structure of the molecule, including its bonds.” Because environmental temperatures cause too many vibrations of the atoms themselves, the experiment must be run in a vacuum at -268C.
The ability to work with single molecules is on the rise. Related work at the nanoscale from Caltech has recently led to the development of a scale that weighs one molecule at a time. Other researchers continue to explore molecular assemblies that can function as their macroscopic counterparts, such as a single-molecule electric motor and even a car developed last year.
Seeing how far the IBM research alone has come in just a few years, the future of this “extreme microscopy” could empower scientists to unravel the properties of many more systems of interest to materials science and nanotechnology. The anatomy of molecules long relegated to the realm of quantum mechanics could be thoroughly explored experimentally, and that is a huge step toward an era of molecular machines. We take for granted the ability to manipulate, inspect, and test objects on our scale, but in order to engineer real machines at the nanoscale, molecular components must stand up to the same quality control. This is true for proposed technologies, such as quantum computing and carbon nanotube space elevators.
When it comes to the nanoscale world, it seems scientists are just getting started, but for now, it’s a playground of new discoveries.