When sperm meets egg, life is ignited in a brilliant flash of light.
If you were asked how babies are made in biology class, you’d probably answer with some version of the above dogma. Although most of us have a cursory idea of how conception works, these first few seconds of a human life are actually mind-bogglingly mysterious.
Think about it: two individual cells merge, ensuring complete mutual destruction. Yet somehow, this union triggers an avalanche of events in the new hybrid proto-life form. When the dust settles, a viable embryo rises from the molecular ashes of its parent cells with the complete capacity to form a full-fledged, functional individual.
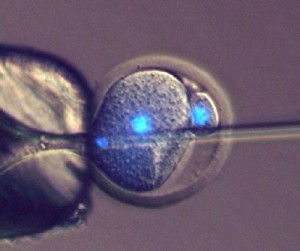
It’s a majestic example of the magic that is human biology, and thanks to a new study published this week in Nature Communications, we have a better idea of how it works.
In a nutshell, a team of British scientist led by Dr. Anthony Perry at the University of Bath used chemicals to transform a mouse egg cell into a bizarre entity called a parthenogenote that behaves somewhat like a fertilized embryo, but with only the mother’s genes.
When scientists injected sperm into the parthenogenotes and implanted them into surrogate mother mice, the human-made embryos grew into normal looking mice pups with a success rate up to 24% — similar to in vitro fertilization (IVF) — which eventually went on to have babies of their own.
Since parthenogenotes mimic the biology of an early-stage embryo, the study shows that life can successfully be made even when sperm are late to the game. That is, even when eggs have already started their unorthodox journey toward forming a new life, sperm cells can still somehow “catch up” to help the process along.
“This is the first time that full term development has been achieved by injecting sperm into embryos,” says Perry in a press release.
Sperm meets egg
To really understand what the team did, let’s zoom in on the first few hours of normal conception.
When a lucky sperm tunnels into an egg, its membrane disintegrates, allowing all the bioactive molecules — paternal DNA, proteins and other molecules — to break free and mingle with the egg’s internal environment.
Unlike the majority of the cells in our body, sperm and egg cells are special in that they only carry half of the complete set of genetic material. This way, when they merge, the resulting embryo has the right amount of DNA to make a healthy baby.
Even the packaging of DNA is different in these reproductive cells. We have a lot of genetic material—stretched out, it’s roughly 6 feet long. To fit it all inside a cell, DNA is tightly wrapped around special proteins somewhat like strings around beads. This bead-necklace-like material is further wound into figure-8 shapes called chromosomes. Both the DNA strand and proteins are often dotted with chemical modifications that change how DNA is expressed (that is, which genes are made into proteins) — this is called the “epigenetic landscape.”
Still with me? Bottom line: when sperms merge with eggs, a kind of “magic sauce” in the egg completely changes the sperm’s epigenetic landscape. The bead-like proteins are swapped by their maternal counterparts; the chemical modifications are wiped. This process is absolutely crucial to transforming sperm’s building blocks into the raw material that makes an embryo.
Normally, this process happens at the time of fertilization (when sperm meets egg).
What the British team wanted to know is how much they can delay the process. What if a sperm meets an egg that’s jumped the gun, already in the beginning stages of dividing into a pseudo-embryo?
Sperm meets parthenogenote
That’s where parthenogenotes (basically, “cell for virgin birth”) come in.
These cells can spontaneously arise from egg cells and behave like early-stage embryos. In lizards, snakes, bees and flatworms, these strictly maternal cells can go on to develop into full organisms — clones — without sperm. In mammals such as mice and men, however, although parthenogenotes sometimes spontaneously arise, the embryos usually shrivel and die halfway through gestation.
The scientists bathed normal egg cells in a chemical concoction for 13 hours, at which point the single-cell parthenogenote was ready to divide into two cells.
They injected sperm into those cells, waited until they finished their division, and selected the cells that received genetic material from both the parthenogenetic embryo and the sperm — in other words, those that got genetic material from both the mom and dad.
When researchers implanted the selected cells into surrogate mothers, roughly a quarter survived to term, producing healthy-looking mouse pups that went on to have pups of their own.
“It had been thought that only an egg cell was capable of reprogramming sperm to allow embryonic development to take place,” says Perry. Since the team produced mice using parthenogenotes, which aren’t strictly eggs, this work challenges that century-old dogma.
Surprisingly, when the team checked the epigenetic landscape of these test tube mice, it was extremely different than that of a normally fertilized one.
“That is, to my mind, really important because it suggests that you can obtain offspring by different epigenetic paths, and that’s the first time, I think, that anyone has said this or suggested this,” says Perry to The Scientist.
Sperm meet non-egg cells?
So what does this all mean?
For one, the very first processes that happen during fertilization could potentially be bypassed without severe consequences. It seems that sperm reprogramming — the resetting of its epigenetic landscape — is far more flexible than scientists had previously thought. Considering that the development of an embryo is a tightly controlled orchestra with little room for error, the observation that an off-rhythm sperm can still lead to live births is pretty amazing.
Also, whatever magic sauce that reprograms sperm in eggs is passed on to parthenogenotes, although we don’t yet know what those factors are. If human parthenogenotes (yes, they exist!) also develop naturally after injecting with sperm, we could potentially have another source of lab-made stem cells.
Here’s where it becomes complete speculation.
Parthenogenotes behave somewhat similarly to non-reproductive cells in our body. As the authors put it, the fact that pseudo-egg cells can reprogram sperm “blurs functional distinctions” between the different cell types (reproductive, non-reproductive, embryonic) in our body.
If there was a way to strip non-reproductive cells — say, skin cells — of half of their genetic material, it may be possible to chemically make them into parthenogenote-like cells, which may in turn develop into full animals (or humans) after injecting with sperm.
Yes, that’s a lot of “mays,” and for good reason. Although there’s been a recent explosion in methods transforming one type of cell into another through different chemical concoctions, stripping a skin cell — or any other type of non-reproductive cell with all 23 pairs of chromosomes — of half its genetic material to form an egg-like cell is entirely unheard of. Getting the transformed cells to express the egg’s reprogramming “secret sauce” is another problem altogether.
That said, with the blazingly fast progress that’s happening in genetic engineering, it’s hard to wholly discount the idea from the realm of possibility.
“Will we be able to do that? I don’t know. But I think, if it is ever possible, one day in the distant future people will look back and say this is where it started,” says Perry.
Image credit: Shutterstock